Cosmology and gravitation
Cosmology is the branch of astrophysics that studies the origin and dynamics of the Universe as a whole. Although the idea of a cosmos sets its roots in human history, cosmology has entered the domain of exact sciences only about 100 years ago.
The emergence of modern cosmology
In the early 1920s, scientists knew that our solar system was located in a galaxy, i.e. a system of billion of stars gravitationally bounded to each other. However, it was unknown whether other objects similar to our own galaxy (the Milky Way) existed.
In 1923, Edwin Hubble realised that the astrophysical object that today we know as the Andromeda Galaxy was indeed a distant source composed of billions of stars, very much like our own Milky Way. Since then, astronomers have observed an ever growing number of galaxies.
In 1929, Hubble made a second ground-breaking observation: he noticed that all the galaxies that he observed were recessing from us [1]. He was able to determine that these galaxies were receding from each other with a velocity directly proportional to their distance:
Fig. 1 : First measurements of the expansion of the Universe by E. Hubble. On the horizontal axis we can see the distance of the galaxies, while on the vertical axis we can see their recession speed. Other galaxies are moving away from each other more rapidly.
v=H0 * d (Eq. 1), où la constante reliant la vitesse et la distance de la galaxie, H0 est appelée constante de Hubble.
Despite this ground-breaking discovery by Hubble, the idea of an expanding Universe was not totally unexpected. In fact, between 1917 and 1922, W. De Sitter and A. Friedman showed that, under the hypothesis of a homogeneous and isotropic Universe, Einstein’s general relativity theory allowed for its expansion [2] [3].
It was immediately recognised that Hubble’s observations could be explained with Einstein’s general relativity: galaxies are not moving away from each other, it is the fabric of the Universe itself, the spacetime, that is expanding
This marked the birth of modern cosmology. Since then, scientists have rapidly advanced in their observation of the cosmos. We have learned that the Universe was born about 13 trillion years ago, that in its early stages it was a dense and hot plasma, and we have observed its fingerprint, The Cosmic Microwave Background (CMB)
Despite our rapid advances in modern cosmology, we are still confronted with crucial open questions that challenge our knowledge of physics. In 1998, it was observed that the Universe is not only expanding, but it is accelerating. According to Einstein’s theory of general relativity, this phenomenon can be explained with the presence of an unknown form of energy which embeds roughly 70% of the total energy of the Universe. This component is called Dark Energy. But what is the nature of Dark Energy? Is is really a new form of energy or does general relativity ceases to be valid at cosmological scales?
Moreover, there are also observational challenges. Our model predicts that the Hubble constant is constant everywhere. However, two independent experiments, based on the observation of the CMB [4] and of our local Universe [5], find two values for H0 that are incompatible with each other. Why do these observations differ? Is there a bias in the experimental setup or are we actually observing a basic difference in the laws of physics at local and cosmological scales?
Gravitational waves
Gravitational waves might help solve cosmology’s open questions. Gravitational waves are perturbations in the fabric of spacetime which propagate at the speed of light. Gravitational waves are very special, since they do not interact with matter during their travel, i.e. they arrive to us through cosmological distances unchanged. From their detection we can directly infer the distance of the source d (see Eq. 1). This is a crucial information for cosmological measurements.
However, this type of radiation is very weak and its detection has represented a major challenge. The first detection was finally achieved only recently, in 2015, with a signal produced by the coalescence of two black holes. In 2017, combining the detection of gravitational waves from a binary neutron star merger with observations of electromagnetic waves (light), the source’s host galaxy could be identified. It represented a major break-through, as it allowed us a completely new measurement of the Hubble constant. The inferred value was 70+12-8 km/Mpc s. This measurement will become more and more accurate as we combine more gravitational wave detections.
Gravitational wave studies at the APC laboratory
At the APC laboratory, we study how gravitational waves can help solve issues in modern cosmology. In particular, we investigate the level of accuracy that can be reached with future gravitational wave detectors in the measurement of the Hubble constant. In fact, the main limitation at present is represented by the precision in the source’s luminosity distance measurement. In order to improve on this parameter’s estimation, our detectors need to effectively detect the two polarizations of gravitational waves, i.e. the directions in which the gravitational wave oscillates. Unfortunately, our detectors are not sensitive to the two polarizations at the same time. For this reason, multiple gravitational wave detectors are needed to localise a signal.
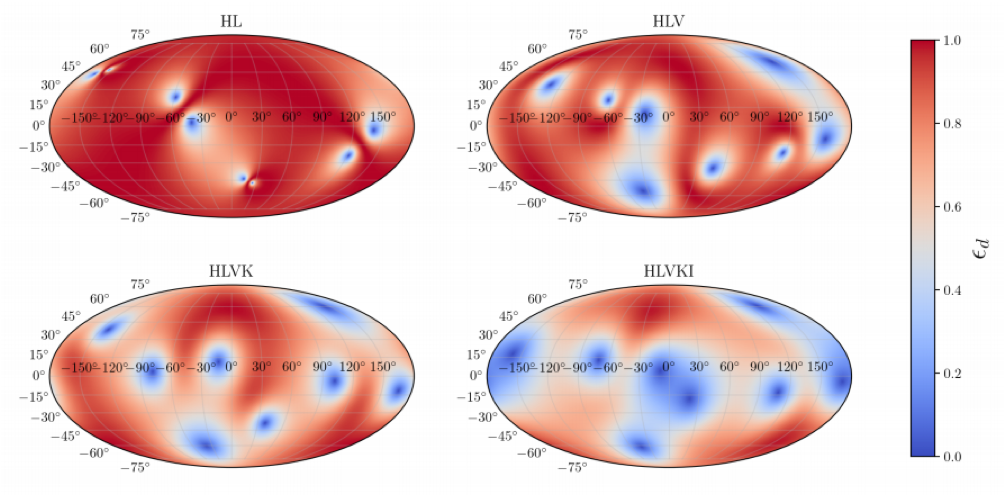